Imagine that you buy a pair of new shiny leather shoes at a shop. However, they are not made of animal leather, but, rather of mycelium filaments. When you step in a puddle wearing your new shoes, their surface will reject water. With use, the shoes will be scuffed and wear out, but that does not matter because their material will repair itself. In the future, this may be very real - using biobased smart materials.
What are smart materials and what can be expected of them in the coming decades?
A smart material reacts to different conditions
Smart watches, smart lamps and smart household appliances. In recent years, different smart applications have infiltrated every aspect of our everyday lives. Smart biomaterials, however, are not about information technology, but, rather, about using the properties found in nature.
What makes a material smart is its responsiveness: it can change by responding to different conditions, such as pressure, light, humidity or temperature. In practice, a component, such as a polymer, is added to the material, the properties of which then change according to external conditions.
The smartness of natural materials stems from enzymes, other proteins or cells that react to external conditions, and thus change the properties of the material. By mimicking such biological mechanisms, the aim is to develop new biobased smart materials. For example, when exposed to oxygen and water, leather shoes made of a smart material could generate an ingredient that repairs the broken spot. For the time being, the development of microbial-based leather still aims to mimic the properties of animal-derived leather as closely as possible. Adding smart features will be included in the next steps of development.
Nature-inspired materials will be biobased and smart in the future
Intelligent materials can be roughly divided into two categories: materials that mimic nature and those in which solutions found in nature are applied in a new way.
For a long time, people have drawn inspiration from nature, copied its structures and properties and applied such findings to the manufacture of materials. For example, the textile industry has developed a material that mimics the structure of a conifer cone, making the textile waterproof or moisture permeable. When wet, a cone will close; when dry, it will open. The designers of a self-cleaning material, which for example can be used in solar panels or on skyscrapers, has also drawn their inspiration from nature. The material mimics the surface of a lotus leaf, which is so water-repellent that water will bead up and then roll down off the petals in small droplets, cleaning the petals.
The features found in nature are also used in a new way in various man-made applications. For example, a VTT research team has developed an impact-resistant nanocomposite in collaboration with a university from Singapore, the structure of which was copied from the dactyl club of the mantis shrimp. Their limbs have a soft shock absorbing inner layer and a hard, shock-resistant outer layer, enabling them to have the strongest impact in the entire animal kingdom. The VTT team produced a similar structure and compiled a new composite made of birch nanocellulose and a protein tailored for this particular composite. For example, tougher and lighter dental implant crowns have been made of the nanocomposite, making it suitable for future products where the material must withstand wear and tear.
The University of Oulu has just developed a self-healing material, modelled on the resin-chitin composite structure of the grasshopper’s leg. The material can be cut completely across, with the cut closing in about a minute without requiring no heating or any other external energy source. In the future, it can be used, for example, in gaskets and electronics.
The second category consists of materials to which humans have applied useful functionalities found in biology. Recently, researchers have been particularly interested in adding live microbes to materials. For example, researchers have produced self-healing concrete by adding to it microbes that are resistant to harsh conditions. When a crack develops in concrete and water enters the structures, microbes activate and repair the crack by precipitating calcium carbonate into the cracks. This allows the material to extend the life of the concrete.
However, when utilising living materials, care must always be taken to ensure that microbes do not spread elsewhere and pose a safety risk. This is partly why their development also takes a longer time.
The materials of the future will repair themselves and break down spontaneously
In the future, smart materials can be used to promote the well-being of both the environment and people. For example, the pharmaceutical industry is developing new biological treatments: biosensors detect internal damage to the body, with biomedicines being activated under certain conditions, enabling them to start producing a drug at a controlled rate.
In addition to medicine, smart materials have many applications in the circular economy. Self-repairing materials extend the life of products, saving natural resources and reducing climate emissions as fewer new products are produced.
Smart materials also make recycling easier. For example, in the recycling of plastics and textiles, the problem is often that many different fibres or compounds are mixed into the product that cannot be any longer separated. For example, in the future we could program different compounds to separate from each other or break down under certain conditions, in which case, for example, the different components of a textile could be disentangled. This would facilitate the recycling of materials.
In order to protect nature, materials can also be developed to break down spontaneously under certain circumstances in the future.
At present, huge amounts of plastic waste still end up in nature. Already, plastic-decomposing enzymes have been discovered, but, at present, plastic materials themselves are not smart enough to break down under certain conditions into compounds that do not cause harm to the environment. Perhaps in the future, a bioplastic or plastic-like material will be manufactured in such a way that a certain combination of water and salt will trigger the material's decomposition: in other words, when plastic waste ends up in the sea, the different properties of seawater compared to fresh water will trigger the decomposition process of plastic. Such new plastics designed to be smart do not need to be made from oil; rather, they can be made using biotechnology, for example, with the help of cells.
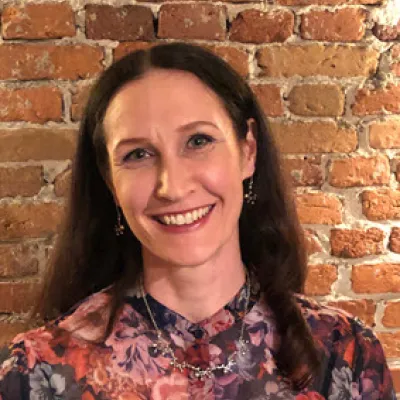