Energy transition, critical materials and geopolitics are becoming increasingly interlinked, and the lack of availability of raw materials is limiting green electrification. Solutions must be sought from sources such as European raw material production, alternative technologies and the circular economy.
The transition to a sustainable energy system will require huge amounts of lithium, cobalt, aluminium and around 30 other critical raw materials. Much greater quantities of them will be needed in 2050 than today, and much more than can be produced at current capacity. Some raw materials will even be needed in much greater amounts than the currently known natural resources. Furthermore, mining and processing are concentrated in countries where environmental protection and human rights are severely lacking.
Battle for raw materials is intensifying – requirements of origin pose additional challenges
Europe's green electrification – replacing fossil fuels with renewable electricity to power transport, heating and industry – will significantly reduce the energy sector's raw material needs, especially as coal, oil and natural gas production declines. However, it requires large investments in technologies and a huge variety of raw materials for these technologies compared to the world of fossil fuels. The green transition is also called the transition from fossil fuels to metals. The world is facing increasingly fierce competition for raw materials, and both EU legislation and research are waking up to the complexities of the problem.
The EU's Critical Raw Materials Act lists 34 substances whose sufficiency is a challenge for green electrification. In addition, requirements of origin will be tightened: according to the renewable energy directive (03/2023), 10% of the raw materials consumed in the EU must come from mines in the EU and 20% from recycling by 2030. This is not an easy target to meet, with old mines becoming depleted, new ones taking an average of 16 years to start up, and few mines having been established in the EU so far this century. Another bottleneck can be the lack of raw material processing facilities and expertise.
Example: Copper and aluminium in the electricity grid
The electricity grid needs to be strengthened as decentralised generation increases and the share of electricity in the end use of energy grows. Also, the need increases for a stronger and wider grid to balance the variability in renewable generation.
“The electricity grid infrastructure is also a good example in the sense that it is an indirect investment need: we don't necessarily want more grid infrastructure, but we need it if we want to promote green electrification. The example also highlights that ‘critical materials’ not only mean the most prominent raw materials, such as lithium for the battery industry, but also the availability of base metals,” says Nelli Putkonen, a research scientist at VTT.
For instance, an IEA (International Energy Agency) report estimates that the need for transmission and distribution grid will double by 2040 if we want to meet the sustainability targets. These grids are typically built of copper or aluminium. The doubling of grid length would mean an estimated increase in annual copper demand for electricity grid construction from 5 to 10 million tonnes, and from 9 to 16 million tonnes of aluminium. Due to the need to upgrade electricity grids alone, total annual demand would therefore increase from 25 to 30 million tonnes of copper and from 64 to 71 million tonnes of aluminium1. Demand for copper and aluminium will also rise significantly in other applications, such as the electrification of transport, which will pose additional challenges to the availability of raw materials and could significantly increase their price. Today, about half of copper demand is produced in the EU, but only about 10% of aluminium 4. The raw material for aluminium comes mainly from Guinea, Brazil and Sierra Leone2. In fact, aluminium production alone accounts for as much as 2% of global CO2 emissions.
Substitute technologies and circular economy tools alongside mining
Alongside increased mining activity – especially in Europe – alternative technologies and solutions are needed. Replacement technologies always come with new drawbacks such as higher costs, increased environmental impact, lower efficiency or the use of other critical materials.
“If a substitute technology is not readily available, the whole solution can be replaced by another: in the example of the electricity grid, for instance, by favouring centralised production or moving production closer to consumption. Whatever the means, this will slow down the green transition and increase the price. If the mutual profitability of technologies changes, it will become more difficult to predict the system and uncertainties will increase,” says Putkonen.
Circular economy solutions are also needed to better manage material flows.
“To ensure the availability of raw materials, recyclability should be taken into account already at the design stage, and the lifetime of technologies should be extended to keep materials in high value use for as long as possible. Attention must therefore be paid to maintenance, repair, reuse and remanufacturing possibilities,” says Jyri Hanski, a senior scientist at VTT.
For many technologies, there are not yet commercial processes for the treatment of end-of-life equipment because there is not yet enough end of life equipment. Investing in the reuse of a technology in the absence of a recycling market requires either strong regulation or the belief of technology manufacturers in a cloudy crystal ball.
“A horrifying picture in 30 years' time may be us cursing how we made such stupid wind turbines, solar cells and batteries whose materials can’t be reused or recycled, even though there is demand for them now,” Hanski adds.
Example: Improving the energy efficiency of buildings
The built environment is a major energy user and source of emissions: In Finland and Europe, buildings consume about 40% of total energy and produce about one third of the greenhouse gas emissions associated with energy use. To meet EU targets, energy consumption and emissions from buildings should be drastically reduced – consumption by 14% and emissions 60% respectively by 2030 compared to 2015 levels.4 At the same time, the global building stock is projected to double in 40 years.5
One way to achieve emission reductions is for buildings to switch to renewable energy sources in their energy use. Under the RED II directive, EU countries must require a certain minimum level for this in new buildings and major renovations. Under Finland's upcoming Land Use and Building Act, 38% of the electricity and heat purchased for buildings will have to be renewable.6 The EU target is 49% of total energy use in buildings by 2030.7
“For buildings, renewable energy means not only more solar and wind power, but also switching to heat pump systems, increased utilization of heat recovery systems and, in the Nordic countries, renewable district heating. Many renewable energy solutions contain critical and strategic raw materials: for example, solar panels use silicon, gallium, germanium, copper and nickel. Wind turbines, on the other hand, contain boron, cobalt, manganese and rare earth metals. The EU and national requirements can hardly be met without solar or wind power, and the future scenarios for critical materials are good to keep in mind when aiming for high renewable energy percentages,” says Paula Ala-Kotila, a research scientist at VTT.
3 + 3 recommendations for managing the complexilty
The role of critical raw materials in the energy transition is complex, consisting of dozens of materials to be monitored, dozens of technologies, their possible alternatives with varying life cycles and an uncertain operating environment. Models of critical material flows are difficult to link comprehensively to energy disruption scenarios. However, a combination of models and scenarios would be necessary, as these materials genuinely constrain the energy transition and guide technology choices.
From the point of view of the policies that govern the use of raw materials, it is easy to make certain recommendations:
- adequate funding and support are needed to develop alternative technologies;
- incentives and regulation for Europe's own sustainable and responsible raw material production and manufacturing industry are needed; and
- more long-term circular economy solutions and better modelling of the future are needed.
Contradictions must, however, be acknowledged: a stricter approach to the increased share of critical raw materials and processed materials sourced from Europe will in the short-term lead us down a path where increasing renewable capacity will be more expensive and slower. Further regulation also puts a strain on the market economy and makes forecasting more difficult. Moreover, while globally it is important to situate mines where they can be operated in a way that respects the environment and human rights, in Europe this will inevitably mean increased damage to local environments.
Keeping complexity, contradictions and an uncertain operating environment in mind, the following must therefore be added to the recommendations for action:
- a wise balancing act between the extremes of the above contradictions, based on a comprehensive sustainability assessment;
- focusing support in particular on robust solutions, i.e. methods and technologies that can operate in a wide range of environments; and
- sufficient resources for research to ensure that as many tools as possible are in the toolbox to tackle the challenges that will inevitably arise.
Statistical sources
KU Leuven (2022) Metals for Clean Energy. (https://eurometaux.eu/media/jmxf2qm0/metals-for-clean-energy.pdf)
Michaux, S. (2021) Assessment of the Extra Capacity Required of Alternative Energy Electrical Power Systems to Completely Replace Fossil Fuels. (https://tupa.gtk.fi/raportti/arkisto/42_2021.pdf)
Information boxes
1 IEA (2022) The role of critical mineral in clean energy transitions. https://iea.blob.core.windows.net/assets/ffd2a83b-8c30-4e9d-980a-52b6d9a86fdc/TheRoleofCriticalMineralsinCleanEnergyTransitions.pdf
2 SCRREEN (2023) Factsheets - CRMS 2023. https://scrreen.eu/crms-2023/
4 European Commission. COM (2020) 662 final. A Renovation Wave for Europe - greening our buildings, creating jobs, improving lives, see. SWD(2020) 550 final. EUR-Lex - 52020DC0662 - EN - EUR-Lex (europa.eu)
5 Why The Building Sector? – Architecture 2030 https://architecture2030.org/why-the-building-sector/
6 Ministry of the Environment (2021) (only in Finnish) https://ym.fi/-/rakennusten-kayttamasta-energiasta-38-prosenttia-oltava-jatkossa-uusiutuvaa
7 Council of Europe. Infographic - Fit for 55: how the EU plans to boost renewable energy (updated 2023) https://www.consilium.europa.eu/en/infographics/fit-for-55-how-the-eu-plans-to-boost-renewable-energy/
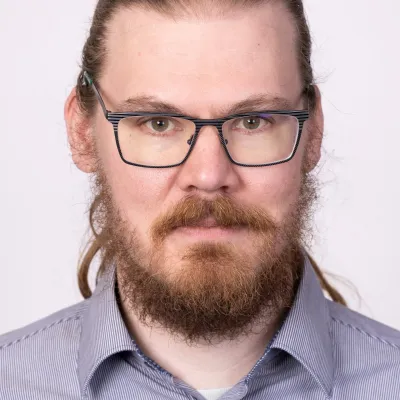
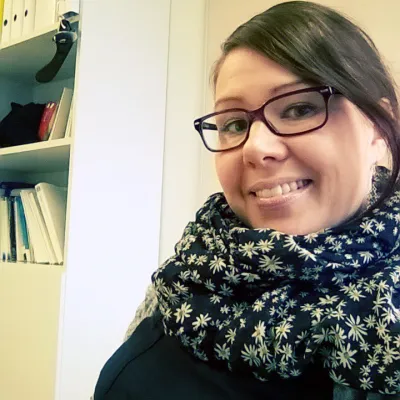
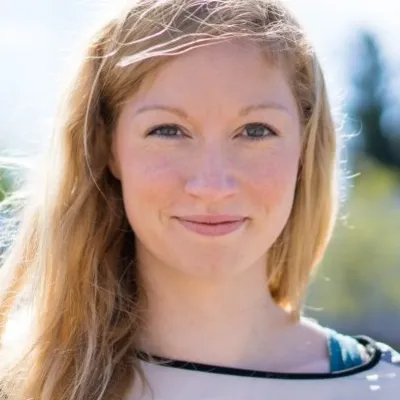